The most notable, and perhaps last, global freezing event occurred approximately 650 to 635 million years ago during the Marinoan Epoch glaciation toward the end of the Cryogenian period (850 to 635 million years ago) in the Neoproterozoic era (approximately 1 billion to 542 million years ago). Some researchers, including a team led by Huiming Bao, Charles L. Jones professor in geology and geophysics at Louisiana State University, contend that this last period of extensive glaciation and the subsequent climate changes might have given rise to modern levels of atmospheric oxygen. This set the stage for the rise of animals and the later explosive diversification of life forms during the episode called the Cambrian explosion.
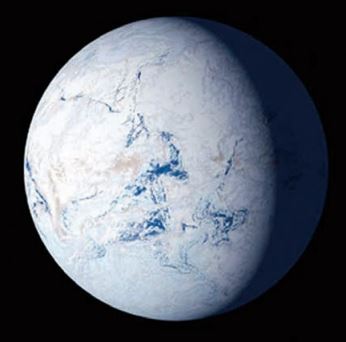
The Snowball Earth concept posits that, during these exceptional cold events, the atmosphere was prevented from warming because most of the incoming solar radiation was reflected back into space by the whiteness of ice and snow covering the oceans and land surface. A lack of heat-retaining clouds and atmospheric moisture, caused by water vapor freezing out of the atmosphere, further amplified this effect. This resulted in average equatorial temperatures of about minus 10 degrees Fahrenheit, roughly similar to presentday conditions in Antarctica. Without the moderating effect of open oceans, the Earth’s climate became much like that of Mars. However, due to some atmospheric sublimation (ice to vapor) and condensation, snow would continue to fall, glaciers would thicken and flow, and sedimentary deposits of glacial origin would be left behind as geological evidence of these severe conditions.
In a January 2000 Scientific Americanarticle titled, “Snowball Earth,” the authors Paul F. Hoffman and Daniel P. Schrag describe evidence from extreme icing events that occured as many as four times between 750 and 580 million years ago during the Neoproterozoic era. These icing events continued into the Ediacaran period (roughly 635-542 million years ago). The Ediacaran, the last period of the Neoproterozic, immediately precedes the Cambrian period of the Paleozoic. The Ediacaran period, named after the Ediacara Hills of South Australia, had its status as an official geological period ratified in 2004 by the International Union of Geological Sciences (IUGS), making it the first new geological period declared in 120 years.
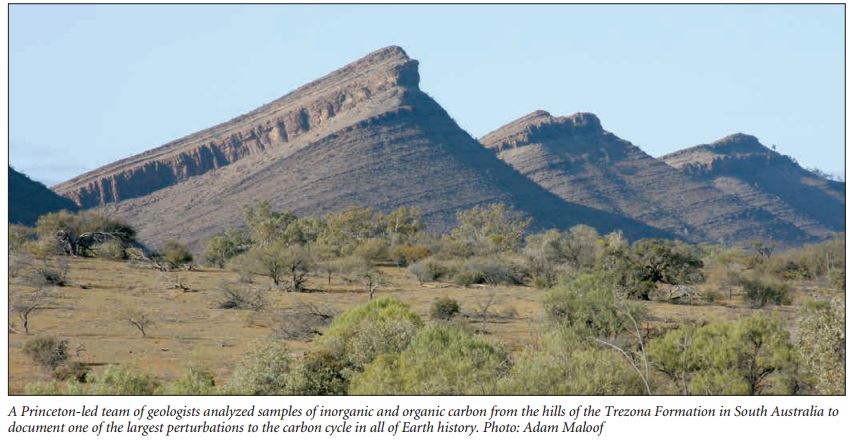 Concept Origins Thick geological deposits hold the only clues to the climate of the Neoproterozoic. For decades, many of those clues were riddled with contradictions. The foremost paradox was the occurrence of glacial debris in sediments deposited near sea level in the tropics. As observed in the January 2000 Scientific Americanarticle, glaciers near the equator today occur only above about 15,000 feet above sea level. Even during the ice ages of the Pleistocene, glaciers reached no lower than 12,000 feet in low-latitude areas. Mixed in with the Neoproterozoic glacial debris are unusual deposits of iron-rich rock which could form only if the oceans and atmosphere contained little or no oxygen, even though by that time the atmosphere had already evolved to nearly the same mixture of gases as it has today. To confound matters, thick carbonate rock sequences, typical of deposition in warm seas, were observed to have been deposited on glacial deposits shortly after the ice receded.
Many geologists have grappled with these puzzling low-latitude glacial deposits. One of the earliest was the Australian geologist and Antarctic explorer Douglas Mawson (1882–1958). Sir Mawson, a Fellow of the Royal Society, on the first team to ascend Mount Erebus, Antarctica’s tallest peak, and on the first team to reach the magnetic South Pole, spent much of his career studying the Neoproterozoic stratigraphy of South Australia where he identified thick and extensive glacial sediments. Late in his career, he speculated about the possibility of global glaciations. However, his ideas of global glaciation were hampered by the mistaken assumption that the geographic positions of Australia, and all the other continents were fixed in their present positions. Not until the development of the plate tectonic theory could geologists see the whole picture of lowlatitude glacial deposits.
In 1964, the idea of global-scale glaciation reemerged when W. Brian Harland (1917 – 2003) published the paper “Critical evidence for a great infraCambrian glaciation” in the International Journal of Earth Sciences. In the paper, Dr. Harland presented paleomagnetic data showing that glacial tillites in Svalbard and Greenland were deposited at tropical latitudes. Based on the paleomagnetic data and the sedimentological evidence of glacial deposits interrupting successions of carbonate rocks commonly associated with tropical to temperate latitudes, he argued for an extreme ice age affecting the tropical regions.
Also in the 1960s, Mikhail Budyko (1920 – 2001), a Russian climatologist at the Leningrad Geophysical Observatory, developed an energy-balance climate model to investigate the effect of ice cover on global climate. Using this model, Dr. Budyko found that if ice sheets advanced far enough beyond the polar regions, to roughly 30 degrees of latitude, a feedback loop developed due to the increased reflectiveness of the ice and snow. The reflectiveness of ice and snow is known as albedo (see the text box). The increased reflectiveness returns the incoming solar energy into space and prevents the Earth’s surface from warming. This leads to further cooling and the formation of more ice, until the entire Earth was enveloped in ice and stabilized in a new ice-covered equilibrium in a run-away icehouse effect. While Dr. Budyko’s model suggested that this shift to an ice-albedo stability could happen, he concluded that it had never happened, because his model offered no way for the Earth to escape from scenario of perpetual deep freeze.
The moniker “Snowball Earth” was coined by Joseph Kirschvink, a professor of geobiology at the California Institute of Technology, in a paper titled “Late Proterozoic low-latitude global glaciation: the Snowball Earth” published in a multidisciplinary study by the Cambridge University Press in 1992. The major contributions from this work were: (1) recognition that the presence of banded iron formations is consistent with a glacial episode where the atmosphere is depleted in oxygen and (2) introduction of a mechanism with which the Earth could escape from an ice-covered condition. This mechanism is the increasing accumulation of atmospheric carbon dioxide (CO2) from volcanic outgassing leading to an ultra-greenhouse effect.
The Snowball Earth hypothesis was brought to widespread attention in the scientific community by the publication of “A Neoproterozoic Snowball Earth,” by Paul F. Hoffman, Alan J. Kaufman, Galen P. Halverson, and Daniel P. Schrag in the August 1998 issue of the journal Science. Dr. Hoffman, professor of geology at Harvard University, and his coauthors studied carbon isotope anomalies in carbonate rocks bracketing Neoproterozoic glacial deposits in Namibia. These glacial deposits included ice-rafted dropstones in equatorial marine strata. The authors combined their observations related to these anomalies with estimates of thermal subsidence history to develop the conclusion that biological productivity in the surface oceans collapsed for millions of years. They explained the collapse as related to ice-covered seas during global glaciations, that is, a snowball Earth. The authors went on to posit that these glacial episodes ended abruptly when subaerial volcanic outgassing raised atmospheric carbon dioxide concentrations to about 350 times greater than modern atmospheric concentrations. The increased carbon dioxide concentrations resulted in a rapid warming due to extreme greenhouse conditions. Additionally, the transfer of atmospheric carbon dioxide to the ocean resulted in the rapid precipitation of calcium carbonate in warming surface waters, producing the carbonate rocks observed globally capping the Neoproterozoic glacial deposits.
How did it Happen? While geological evidence of Neoproterozoic glaciations is strong, a thorough understanding of the mechanisms that caused the extreme temperature plunge is incomplete. Current thinking is that a reduction in the concentration of “greenhouse” gases, particularly carbon dioxide and methane, led to a globally colder climate. The colder climate allowed areas of ice to expand from the polar regions into lower latitudes. Researchers theorize that when the earth became approximately 50 percent covered by ice, the global climate reached a tipping point where the solar radiation was no longer able to warm the Earth surface due to the increased albedo leading to a runaway icehouse effect.
But what caused the lowering of atmospheric concentrations of the greenhouse gases? CO2 cycles through the atmosphere and ocean starting with volcanic emanations and ending with removal as calcareous sediments and organic matter. In the atmosphere, CO2 forms a weak carbonic acid with precipitation. After falling on land, the slightly acidified precipitation is neutralized by the weathering of silicate rocks, a process particularly active in warmer climates. These processes remove carbon from the atmosphere and transfer it to the world’s oceans as bicarbonate ions. The bicarbonate is removed from the oceans primarily by biological activity as calcium carbonate. The calcium carbonate settles to the bottom and enters the lithosphere.
Paleomagnetic data indicate that during the Cryogenian period, encompassing the Sturtian and Marinoan glaciations, most of the continental landmasses were located in the Earth’s warmer equatorial regions. This suggests that the rate of silicate weathering was high, lowering atmospheric CO2 concentrations, slowing greenhouse heating, and cooling the global climate. The global cooling lowered the silicate weathering rate, ultimately stabilizing the climate system at a new colder state.
Two additional phenomena are known to have occurred at the time, further contributing to high silicate weathering rates and lower CO2 concentrations. The first was the breakup of the pre-Pangean supercontinent named Rodinia which began approximately 830 million years ago and continued for nearly 200 million years. Silicate weathering rates are thought to be low when a supercontinent exists because most land area is far from the ocean and therefore very dry. When a supercontinent breaks up, formerly arid regions become wetter increasing weathering rates and reducing atmospheric CO2 concentrations.
The second phenomenon was the massive eruption of flood basalts around 723 million years ago in the present-day Canadian arctic which then lay close to the equator. Basalts weather rapidly and are a rich source of calcium ions. Model simulations published in the a February 2004 letter to the journal Naturetitled “A ‘snowball Earth’ climate triggered by continental break-up through changes in runoff” by Yannick Donnadieu, Yves Goddéris, Gilles Ramstein, Anne Nédélec, and Joseph Meert indicate that the combined effects of equatorial continents, supercontinent breakup, and low-latitude flood basalt emplacement are sufficient to cause a depression of atmospheric CO2 concentrations and trigger a global glaciation episode.
Survival of Life An ice-covered Earth would curtail photosynthetic life on Earth and thus drastically deplete atmospheric oxygen. Detractors argue that this kind of glaciation would have made life extinct entirely. However, microfossils such as stromatolites and oncolites prove that, in shallow marine environments at least, life did not suffer any significant disruption. Proponents theorize that life could have survived or even flourished in the following locations:
- Deep oceanic hydrothermal vents.
- Under the ice in chemolithotrophic (mineral-metabolizing) ecosystems theoretically resembling those in existence under modern glaciers.
- Small regions of deep, open, ice-free ocean far from the supercontinent Rodinia, where photosynthesizers could have access to light and CO2.
- On high mountain areas in the tropics where daytime tropical sun or volcanic heat could create small temporary melt pools.
- In pockets of liquid water within and under the ice caps, similar to Lake Vostok in Antarctica.
- In small oases of liquid water near geothermal hotspots resembling Iceland today.
A continuing mystery is that the fossil record indicates that organisms and ecosystems do not appear to have undergone the significant change that would be expected by an extreme climate event or a potential mass extinction associated with the global glaciations. Even if life were to cling on in all the ecological refuges listed above, a whole-Earth glaciation should result in a biota with a noticeably different diversity and composition. This change in diversity and composition has not yet been observed in the fossil record — in fact, the organisms which should have been most susceptible to climatic variation emerge unscathed from the Snowball Earth.
Escape from Snowball Earth Climate models, such as those developed by Dr. Budyko, suggest that once the Earth is covered in ice there would be no escape. Solar radiation, largely reflected by the glittering white surface, would be insufficient to loosen the icy grip. Yet, our currently ice-free tropics and temperate world indicate that the atmosphere warmed sufficiently to end the glacial event. Geological evidence indicates that elevated levels of CO2 concentrations in the atmosphere from volcanic outgassing led to rapid warming. Atmospheric concentrations of CO2 during a period of global glaciation would continue to increase because there were no open ocean surfaces to absorb the gas and no precipitation to remove it.
As advanced in the paper “CO2 levels required for deglaciation of a ‘near-snowball’ Earth,” by the authors Thomas J. Crowley, William T. Hyde, and W. Richard Peltier from the Department of Oceanography, Texas A&M University, published in the January 2001 Geophysical Research Letters of the American Geophysical Union, the CO2 levels necessary to unfreeze the Earth are estimated to have been about 130,000 parts per million, or 13% of the atmosphere. Their research indicates that, over 4 to 30 million years, enough CO2 and methane, mainly emitted by volcanoes, would accumulate to finally cause a strong enough greenhouse effect to lead to surface ice melting in the tropics. Eventually, an equatorial band of permanently ice-free land and water developed. Methane trapped in equatorial permafrost would be released adding to the greenhouse warming. The newly-exposed ocean and ground would be darker than the ice, and thus absorb more energy from the sun — initiating a “positive feedback” furthering the global meltdown.
On the continents, the melting glaciers would release massive amounts of water and glacial sediment flowing to the oceans. The resulting sediments supplied to the ocean would be rich in mineral nutrients which, combined with the abundance of CO2, would trigger a cyanobacteria population explosion, causing a relatively rapid re-oxygenation of the atmosphere. Researchers suggest that this vastly different oxygen-rich world was the setting for the rise of the Ediacaran biota and the subsequent Cambrian explosion. This positive feedback loop of mechanisms would free the Earth from ice in an astonishingly short period of time, perhaps less than 1,000 years. However, replenishment of atmospheric oxygen and depletion of the CO2 levels by ocean absorption and biological uptake would take further millennia.
Conclusion Any theory that describes a radical departure from conventional models is sure to have critics. The Snowball Earth concept is no exception. The great antiquity of the Neoproterozoic glacial era deposits makes determinations relative to timing, global extent, and duration difficult and subject to uncertain interpretations in this developing area of study. Yet the geologic evidence for the occurrence of equatorial glaciations at this time is not disputed. However, the mechanisms, triggers, and processes that could lead to an ice-covered Earth continue to draw much debate. Does the Earth’s climate have a tendency to shift to a colder state? “Indeed, one of the great puzzles is why hasn’t this extent of global freezing happened since the Proterozoic,” said Jerry McManus, paleoclimatologist and professor of geological sciences at Columbia University’s Lamont-Doherty Earth Observatory in New York. Some scientists theorize a less severe Snowball Earth scenario that they term “Slushball Earth,” where the oceans were covered in a loose icy mush with large areas of open water rather than a rigid frozen surface.
But one thing is certain, faced with future Snowball Earth conditions, salt supplies at the hardware store would run out very quickly. “We’re gonna’ need a bigger snow shovel.”
|